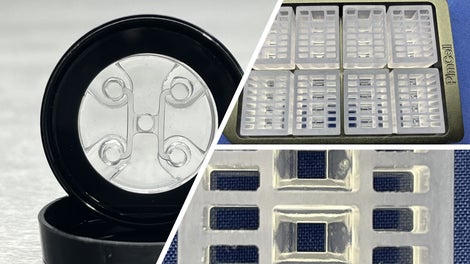
Microfluidic systems are at the heart of cutting-edge research in biological, chemical, and pharmaceutical sciences, powering innovations like organ-on-a-chip technology, drug development, and high-throughput screening. A key component of these systems is the microfluidic chip, which houses tiny chambers and channels designed to precisely control fluid movement at the microscale. The design of these chips is critical for optimizing their performance, with a central debate revolving around whether square or round surfaces are better for accommodating microfluidic units.
While both square and round chips have their place in specific applications, square surfaces often emerge as the more efficient option. Square designs allow for more efficient use of space, enabling higher densities of microfluidic units, which translates into increased throughput and capacity. This post will explore why square microfluidic chips tend to outperform round ones, especially when integrated into applications such as organ-on-a-chip technology, tissue engineering, and large-scale biological assays.
The Efficiency of Square Surfaces
In terms of spatial efficiency, square surfaces make better use of the available area than round surfaces. This comes down to basic geometry: square designs eliminate the underused or wasted space often found at the curved edges of round chips. Microfluidic units on a square chip can be arranged in a grid-like pattern, allowing for precise organization and packing. This is critical in high-throughput applications, where the aim is to maximize the number of experiments or processes running simultaneously.
In contrast, round chips create ‘dead zones’ near the edges, where fewer microfluidic units can be accommodated. This leads to a reduction in the total number of functional units that can be integrated onto the chip, making round designs less ideal for applications requiring high density and efficiency.
Furthermore, square surfaces provide straight edges that allow microfluidic channels and chambers to fit closely against one another. This close packing increases the capacity of the chip and allows for more efficient use of materials and space.
Organ-on-a-Chip Technology and the Role of Square Chips
One of the most exciting and rapidly evolving applications of microfluidic systems is organ-on-a-chip technology. These devices mimic the structure and function of human organs, providing a controlled environment for studying biological processes, disease mechanisms, and the effects of drugs on human tissues. The success of organ-on-a-chip technology heavily relies on the design and layout of the microfluidic chips used.
Square microfluidic chips are particularly well-suited for organ-on-a-chip systems. The grid-like organization of microfluidic units on a square chip allows for precise control over fluid flow, which is essential for simulating the microenvironments of organs. These chips can be designed to support different organ tissues, including lung, liver, heart, and kidney models, enabling researchers to study organ-specific functions in detail.
For instance, in a lung-on-a-chip system, the square design allows for the integration of microchannels that mimic the airways, facilitating the study of respiratory functions, gas exchange, and drug responses. Similarly, in a liver-on-a-chip system, the square layout can support a higher density of hepatocyte cultures, providing more data in a single experiment compared to a round chip.
Square microfluidic chips also enable better scalability for organ-on-a-chip platforms. Researchers can easily add more organ models to a single chip, creating multi-organ-on-a-chip systems that allow for the study of interactions between different organs, such as the liver and kidney. This approach opens new avenues for understanding complex biological interactions and for testing drug effects across multiple organ systems simultaneously.
Key Benefits of Square Microfluidic Chips
The specific advantages of square-shaped microfluidic chips go beyond just space efficiency. Here are several key benefits:
- Space Efficiency
Square microfluidic chips utilize surface area more effectively than round chips. The straight edges of a square layout allow for tight packing of microfluidic units, channels, or chambers, eliminating the underutilized space that occurs in circular designs. This feature is particularly important for applications like organ-on-a-chip, where maximizing the functional area is essential.
- Higher Density of Microfluidic Units
By eliminating 'dead zones,' square surfaces can accommodate a higher density of microfluidic units. This means that more tests, processes, or experiments can be performed simultaneously, increasing throughput and efficiency. In applications like organ-on-a-chip, this leads to better simulation of biological systems by providing more data points for each organ model.
- Increased Throughput
Square microfluidic chips provide a significant advantage in high-throughput applications, where time and cost are critical. By accommodating more units on a single chip, square designs allow for more parallel processes, leading to faster data collection and cost savings. This is particularly beneficial in organ-on-a-chip studies, where researchers need to run multiple experiments in parallel to test drug efficacy or study disease progression.
- Optimized for Automation
The grid-like layout of square chips is ideal for automated systems commonly used in high-throughput screening and organ-on-a-chip applications. Robotic systems can easily handle square grids for tasks like liquid handling, sample loading, and data collection. This further enhances the efficiency and scalability of microfluidic workflows, making square chips the preferred choice for researchers using automated systems.
- Versatility in Design
Square microfluidic chips offer greater flexibility in design, allowing for the customization of microfluidic units to fit specific needs. For example, researchers can create chips with multiple compartments for different organ models, allowing for the simultaneous study of organ interactions. This versatility is crucial for advanced organ-on-a-chip platforms, where the complexity of biological systems requires precise control over fluid dynamics and spatial organization.
Applications in Organ-on-a-Chip Technology
Square microfluidic chips play a vital role in advancing organ-on-a-chip technology, enabling researchers to better simulate human organs in vitro. Some key applications include:
Lung-on-a-Chip: Square chips allow for the integration of microchannels that simulate the airways and alveolar structures, providing a platform for studying respiratory functions, gas exchange, and drug delivery to the lungs.
Liver-on-a-Chip: The dense packing of microfluidic units on a square chip supports large-scale hepatocyte cultures, making it ideal for studying liver metabolism, drug toxicity, and disease models like non-alcoholic fatty liver disease (NAFLD).
Heart-on-a-Chip: Square chips can be designed to support cardiac tissue cultures, enabling researchers to study the effects of drugs on heart function, electrical activity, and tissue regeneration.
Kidney-on-a-Chip: By mimicking the nephron structure, square microfluidic chips provide a controlled environment for studying kidney filtration, drug clearance, and disease progression in conditions like chronic kidney disease (CKD).
Multi-Organ-on-a-Chip: Square chips offer the flexibility to integrate multiple organ models into a single platform, enabling the study of organ interactions. This is particularly useful for assessing how drugs or diseases affect different organs in tandem, providing a more holistic view of human biology.
Challenges with Round Chips
While round microfluidic chips are still used in certain applications, they have several inherent limitations. The curved edges of round surfaces create 'dead zones' where microfluidic units cannot be efficiently placed, reducing the overall capacity of the chip. This is a major drawback in high-throughput and large-scale experiments, where maximizing the number of functional units is critical.
Additionally, round chips are often harder to scale up for automated systems. Many robotic systems used for liquid handling and data collection are designed to work with square or rectangular grids, meaning round chips may require custom setups or manual handling, adding complexity and cost to the process.
Conclusion
When it comes to designing efficient microfluidic systems, square microfluidic chips offer distinct advantages over round chips, particularly in applications like organ-on-a-chip technology. The ability to accommodate a higher density of microfluidic units, maximize space utilization, and integrate seamlessly with automated systems makes square chips the preferred choice for researchers aiming to simulate human organs in vitro.
Square chips provide a versatile, efficient platform for a wide range of biological and chemical assays, enabling faster, more accurate results in fields like drug discovery, disease modeling, and tissue engineering. As organ-on-a-chip technology continues to evolve, square microfluidic chips will play an essential role in advancing our understanding of human biology and improving drug development processes.
For more information on advanced microfluidic chip designs, including microfluidic PimCell® chips visit PimCell® or Pimbio.